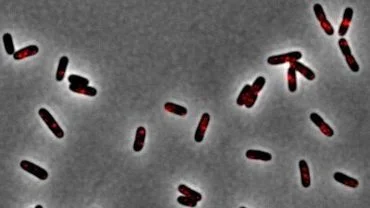
Active areas of research in the Brewster lab
Projects in the Brewster lab share a common theme of combining synthetic and systems biology techniques with simple models to learn the fundamental principles of gene regulation
Characterizing TF function
There are dozens of methods for determining where TFs bind and which genes they regulate. What we lack is a way to systematically characterize WHAT they do when bound. We have developed a method for inferring the regulatory function of TFs acting in isolation. Through a simple model of regulation we are able to infer which steps a TF is acting on and quantify how much a TF acts on each step. Through this process we are learning to characterize the fundamental regulatory role of individual TFs. Fundamental questions that remain unanswered relate to the role of TF identity, TF binding location, operator sequence and promoter identity in setting TF regulatory roles.
Understanding dynamics and timing of transcription networks
There has been a steadfast focus on how the promoter sequence encodes the steady-state response of a gene to a stimulus. Less attention has been paid to how the dynamics of expression are encoded in the promoter. We are focused on understanding how regulatory sequence, network architecture and physiology set the fundamental timescales of gene expression and how these timescales can be manipulated through regulation.
We are using time-lapse microscopy, synthetic biology and computational methods such as stochastic simulations to build simple circuits and predict the relative timing of genes within a network.
The consequence of “overworked” TFs
It has been said that the cell is an efficient machine where evolutionary forces quickly eliminate waste. As a result, one motif that is prevalent in bacteria is that resources are shared. This sharing forces an interconnectivity between otherwise "isolated" cellular functions which must compete for the same required resource. This is especially true in transcription where the different enzymes and transcription factor proteins that perform and control gene expression are required by different, unrelated genes across the genome. This situation is further complicated by DNA substrates that exist in high copy number; Plasmids, viral vectors, and repeated gene segments are all capable of exerting a strong demand on a given transcription factor or transcription associated enzyme and "exhausting" the available supply. The response to this exhaustion is sharp and non-linear and thus can have drastic consequences for the cell. The goal in this project is to gain the ability to quantitative predict these effects as a function of the gene architecture and molecular parameters of that architecture (such as number of binding sites, location of binding sites, copy number of proteins, etc).